Revolutionizing Semiconductor Tech
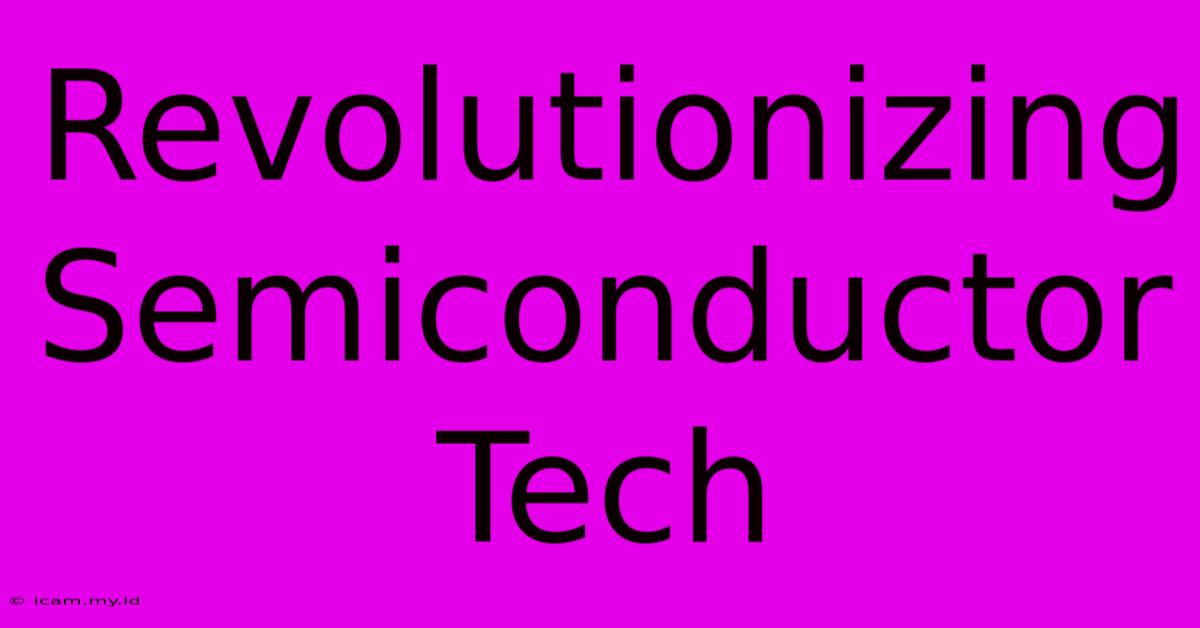
Find more detailed and interesting information on our website. Click the link below to start advanced information: Visit Best Website meltwatermedia.ca. Jangan lewatkan!
Table of Contents
Revolutionizing Semiconductor Tech: The Next Generation of Innovation
The semiconductor industry is the backbone of modern technology. From smartphones and laptops to automobiles and medical devices, semiconductors are everywhere. But the relentless march of technological advancement demands constant innovation, pushing the boundaries of what's possible in semiconductor technology. This article delves into the key areas driving this revolution, exploring the breakthroughs that are reshaping the industry and the implications for the future.
The Drive for Miniaturization: Moore's Law and Beyond
For decades, Moore's Law – the observation that the number of transistors on a microchip doubles approximately every two years – has guided the industry. This relentless miniaturization has led to exponentially increasing computing power and decreased costs. However, the physical limits of silicon are becoming increasingly apparent. As transistors shrink, quantum effects become more pronounced, leading to leakage currents and unreliable operation. This necessitates a multi-pronged approach to overcome these challenges:
1. Advanced Lithography Techniques:
EUV (extreme ultraviolet) lithography is currently the leading-edge technology for creating ever-smaller features on chips. However, even EUV is facing limitations. Research into next-generation lithography techniques, including directed self-assembly and nanoimprint lithography, is crucial for continuing the miniaturization trend. These techniques offer the potential for higher throughput and lower costs compared to EUV.
2. New Materials and Architectures:
Silicon's dominance is being challenged by exploring alternative materials like gallium nitride (GaN) and silicon carbide (SiC). These wide-bandgap semiconductors offer superior performance in high-power and high-frequency applications, making them ideal for electric vehicles, 5G infrastructure, and power electronics. Furthermore, novel architectures like 3D chip stacking are enabling increased density and performance without relying solely on planar scaling.
Beyond Silicon: Exploring Novel Semiconductor Materials
The limitations of silicon are prompting intense research into alternative materials with superior properties. These materials offer the potential to overcome the challenges associated with miniaturization and enable new functionalities:
1. Gallium Nitride (GaN) and Silicon Carbide (SiC):
As mentioned earlier, GaN and SiC are revolutionizing power electronics. Their wide bandgap allows for higher operating voltages and temperatures, resulting in more efficient power conversion and reduced energy loss. This translates to significant benefits in various applications, including electric vehicles, renewable energy systems, and data centers.
2. 2D Materials:
Two-dimensional (2D) materials like graphene and molybdenum disulfide (MoS2) possess unique electronic and optical properties. Graphene's exceptional conductivity makes it a promising material for interconnects, while MoS2's semiconducting properties make it suitable for transistors. However, challenges remain in controlling the properties and scalability of these materials.
3. Perovskites:
Perovskite materials are emerging as a promising alternative for solar cells due to their high power conversion efficiency and low manufacturing costs. Their tunable optoelectronic properties also hold potential for other applications, such as LEDs and sensors.
Advanced Packaging Technologies: Connecting the Dots
Advanced packaging technologies are crucial for integrating multiple chips and components into a single system. These techniques are not only essential for overcoming the limitations of miniaturization but also for enabling new functionalities and improved performance:
1. 3D Chip Stacking:
3D chip stacking allows for the vertical integration of multiple chips, significantly increasing the overall density and performance of a system. This technology is widely adopted in high-performance computing and mobile devices.
2. System-in-Package (SiP):
SiP integrates multiple components, including passive components and chips, into a single package. This approach simplifies assembly, reduces size, and improves performance.
3. Heterogeneous Integration:
Heterogeneous integration combines different types of chips and materials into a single system, enabling the optimization of specific functionalities. This is particularly important for applications requiring a combination of high-performance computing, high-power electronics, and advanced sensors.
Artificial Intelligence and Machine Learning in Semiconductor Design:
AI and ML are transforming the semiconductor design process, accelerating the development cycle and improving the quality of chips:
1. Design Automation:
AI-powered tools are streamlining the design process, automating tasks like circuit design, verification, and optimization. This significantly reduces the time and resources required for developing new chips.
2. Predictive Modeling:
ML algorithms can predict the performance and reliability of chips before they are manufactured, minimizing the risk of costly design errors.
3. Process Optimization:
AI and ML can optimize the manufacturing process, improving yield and reducing defects. This translates to lower costs and faster production times.
The Future of Semiconductor Technology: Challenges and Opportunities
The semiconductor industry faces significant challenges, including geopolitical factors, supply chain disruptions, and the increasing complexity of chip design. However, the opportunities are equally immense:
1. Sustainable Semiconductors:
The industry is increasingly focused on sustainability, exploring eco-friendly materials and manufacturing processes. Reducing the environmental impact of semiconductor production is crucial for the long-term viability of the industry.
2. Quantum Computing:
Quantum computing holds the potential to revolutionize various fields, from drug discovery to materials science. However, the development of commercially viable quantum computers requires significant advancements in semiconductor technology.
3. Neuromorphic Computing:
Neuromorphic computing mimics the structure and function of the human brain, offering the potential for significant improvements in energy efficiency and processing power.
Conclusion:
The revolution in semiconductor technology is an ongoing process, driven by relentless innovation and the pursuit of ever-increasing performance. From advanced lithography techniques and new materials to innovative packaging and AI-powered design tools, the industry is continuously evolving. Overcoming the challenges and harnessing the opportunities will be crucial for shaping a future where technology continues to advance at an unprecedented pace, ultimately benefiting society as a whole. The future of semiconductor technology is bright, promising groundbreaking advancements across countless sectors. The next generation of innovations will be defined by collaboration, sustainability, and a focus on addressing the grand challenges facing humanity.
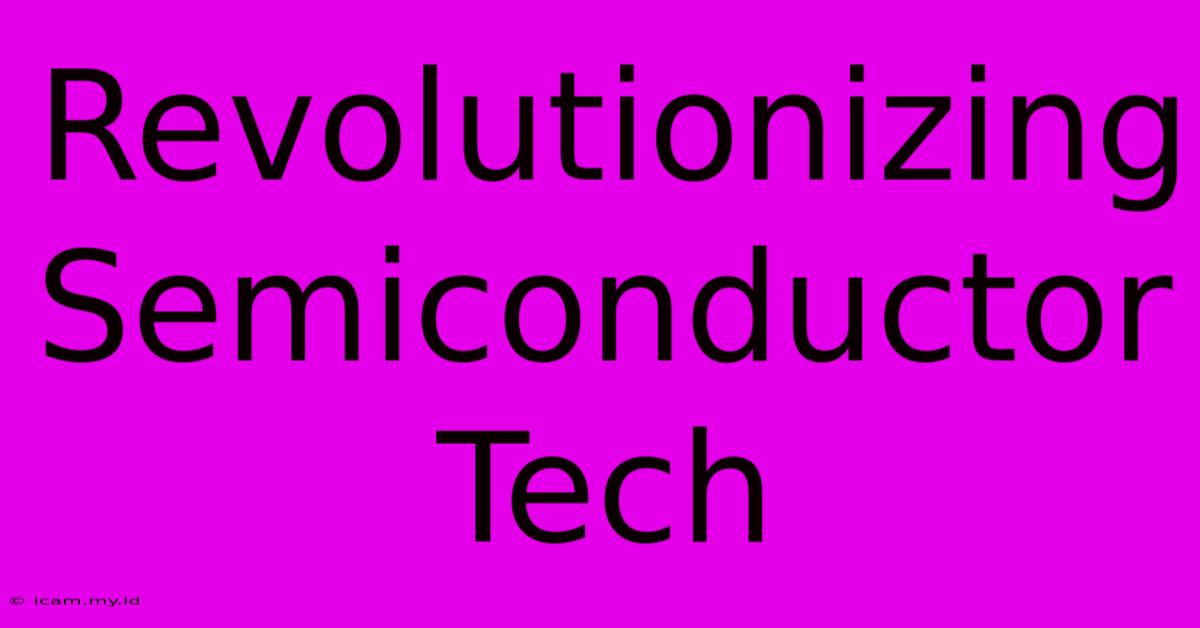
Thank you for visiting our website. Revolutionizing Semiconductor Tech. We hope the information we provide is helpful to you. Feel free to contact us if you have any questions or need additional assistance. See you next time, and don't forget to save this page!
Kami berterima kasih atas kunjungan Anda untuk melihat lebih jauh. Revolutionizing Semiconductor Tech. Informasikan kepada kami jika Anda memerlukan bantuan tambahan. Tandai situs ini dan pastikan untuk kembali lagi segera!
Featured Posts
-
Separate Standards Urgent For Carbon Credit Market
Nov 30, 2024
-
Leicesters Potential Nistelrooy Signing
Nov 30, 2024
-
Kl Rovers Final Piala Malaysia Session
Nov 30, 2024
-
Indonesian Food Program Tech Backing
Nov 30, 2024
-
The Growing Rift Between Marcos And Duterte
Nov 30, 2024